Fruit processing
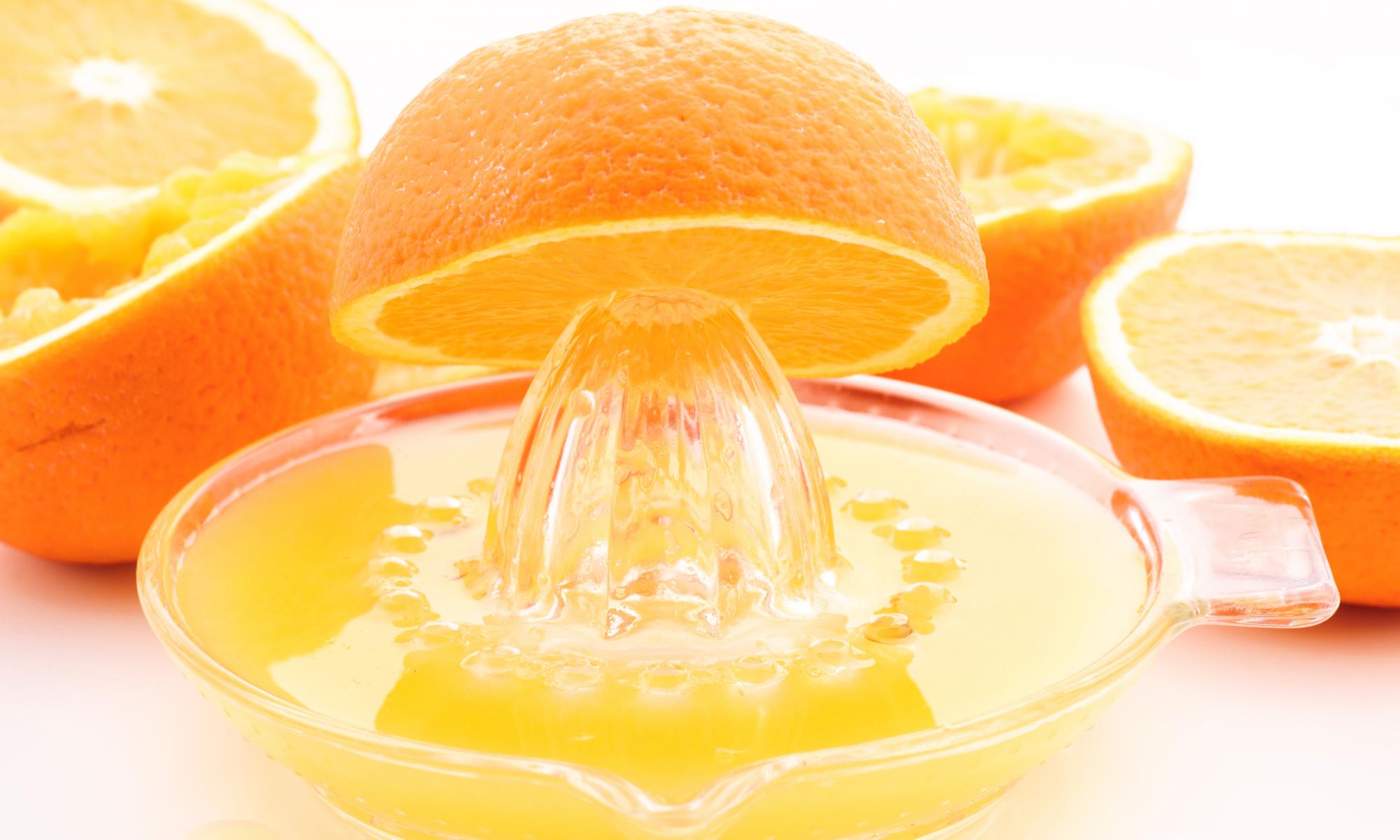
In this chapter you will read about:
- The production steps in a processing plant.
- Fruit delivery and what is done at the fruit reception area to prepare oranges for juice extraction.
- How the maximum amount of juice is squeezed from fruit and the two principal extractor types.
- Removing pulp by clarification so that juice can be evaporated to concentrate or processed to single-strength juice.
- NFC production including heat treatment and bulk storage.
- Concentrate production and the need for efficient evaporators.
- The recovery of peel oil. How winterization makes wax drop out.
- The production of animal feed from waste streams.
- Pulp production and the many uses of pulp.
- Washing of pulp to recover valuable solids.
5.1 Processing plant overview
Orange processing plants are located in the vicinity of the fruit growing area. Fruit should be processed as soon as possible after harvesting because fruit deteriorates quickly at the high temperatures found in citrus-growing areas. Orange products, on the other hand, are produced in a form that allows them to be stored for extended periods and shipped over long distances.
In the orange industry, the basic unit of reporting crop and plant intake is commonly the fruit box.
A box of oranges is defined as containing 40.8 kg (90 lb) of fruit. In Florida, small and medium-sized plants typically process 5-10 million boxes (200,000-400,000 tonnes) per season, the large plants up to 25 million boxes. Most Brazilian citrus plants have much higher capacity. The world’s largest orange juice plant, Citrosuco in Matao, Brazil, can take in 60 million boxes (2.4 million tonnes) of fruit during a season. In most other orange-growing regions, citrus processing plants are considerably smaller than those in Florida and Brazil. Figure 5.1 shows the typical orange processing steps.
Fruit reception
Fruit is delivered in trucks that discharge their loads at the fruit reception area. The fruit may be prewashed to get rid of immediate surface dirt and pesticide residue before any leaves and stems still attached are removed. Then follows pregrading by manual inspection to remove any unsuitable fruit. Sound fruit is conveyed to storage bins. Damaged fruit goes directly to the feed mill.
Extraction
Extraction involves squeezing or reaming juice out of either whole or halved oranges by means of mechanical pressure. After final washing and inspection, the fruit is separated according to size into different streams or lanes. Individual oranges are directed to the most suitable extractor in order to achieve optimum juice yield. As the extraction operation determines juice yield and quality, the correct setting of extractor operating conditions is very important.
Clarification
After extraction, the pulpy juice (about 50% of the fruit) is clarified by primary finishers that separate juice from pulp. The finishing process is a mechanical separation method based on sieving. The juice stream is further clarified by centrifugation. The pulp stream, containing pieces of ruptured juice sacs and segment walls, may then go to pulp recovery or to pulp washing.
Frozen concentrated orange juice (FCOJ) production
From the buffer/blending tanks and after clarification, the juice goes to the evaporator. Within the evaporator circuit, the juice is first pre-heated and held at pasteurization temperature. It then passes through the evaporation stages of the process, where it is concentrated up to 66 °Brix. During the evaporation process, volatile flavour components flash off and can be recovered in an essence recovery unit.
Juice concentrate is cooled and blended with other production batches as required to level out fluctuations in quality. It then goes to frozen storage in tanks or drums as FCOJ, sometimes for several years.
Not-from-concentrate juice (NFC) production
An alternative to concentrate production is to process the juice at single strength as an NFC product. Clarified juice is pasteurized before storage. Deoiling may be required to reduce oil levels in the juice, and deaeration to remove oxygen is part of good practice.
NFC is stored in bulk either frozen or in aseptic conditions. Storage may be up to a year because while consumption is year-round, production is seasonal.
Pulp production
For pulp recovery, pulpy juice from the extractor is passed through a system that removes defects where undesirable pulp components, such as seed and rag, are removed. The clean pulp stream is then concentrated in a primary finisher. After heat treatment, the pulp slurry is typically concentrated further before being sent to frozen storage.
Pulp wash
If the pulp fraction is not recovered for commercial sale, pulp from the final juice finishers and clarifiers can be washed with water to recover juice solubles. This stream is called pulp wash and may, legislation permitting, be blended with juice before concentration.
Peel oil recovery
Recovered peel oil represents some 0.3% of the fruit intake. The emulsion of oil and water from the extractor section is clarified by centrifugation in two steps. The purified oil contains dissolved waxes that are removed by winterization (refrigeration) of the oil for a specific time.
Feed mill
It is economically feasible to include a feed mill operation in larger processing plants. Rejected fruit from grading, peel and rag from extraction, and washed pulp and other solid waste is sent to the feed mill where it is dried and pelletized for animal feed. Smaller plants usually truck their solid waste to a plant with a feed mill or dispose of it in other ways, such as landfill.
5.2 orange juice production steps
All production steps for orange juice, from orange fruit to packaged product, are shown in Figure 5.2. The steps carried out in the fruit processing plant, as highlighted in the diagram, are discussed in further detail during the chapter.
5.3 Fruit reception
After harvesting, fruit picked in the groves is loaded onto trucks (typically 20 tonnes in Florida) and taken to the processing plant. Figure 5.3 shows the subsequent processing flow at the fruit reception.
Truck unloading
The trucks are unloaded onto a specially designed tipping ramp. The ramp lifts the front of the truck to allow the fruit to roll off the rear of the trailer directly onto a conveyor. The fruit is then conveyed to the prewash station. Alternatively, the truck may be reversed down a ramp so that the fruit is unloaded directly onto a conveyor.
Prewashing, destemming and pregrading
The fruit may undergo initial washing to remove dust, dirt and pesticide residues. Many processors have discontinued washing the fruit before bin storage because wet fruit in the bins can make downstream sanitation more difficult. The fruit then moves on to destemming and pregrading.
The roller conveyor of the destemming and pregrading tables allows any leaves or twigs to fall through the conveyor bed. Pregrading by manual inspection removes rotten and visibly damaged fruit. Rejected fruit, known as culls, may be sent to the feed mill.
There is a strong desire to reduce total water consumption in orange processing plants. Water used for prewashing is often condensate recovered from the evaporation process. The condensate is cooled and/ or treated with a sanitizing agent to limit microbial contamination, in particular of Alicyclobacillus.
Sampling
A sample of fruit is taken from each truck for analysis. The main parameters analysed are juice yield, °Brix, acidity and colour. This gives the processor an indication of fruit ripeness. As the fruit goes into bin storage, each load can be tagged and identified. It is then possible to select suitable fruit from various sources for blending during the extraction process to achieve the desired final product quality. The measured juice yield may also form the basis for payment to the fruit supplier.
Fruit storage
The pregraded fruit is stored in bins specially designed with inclined multilevel internal baffles. These distribute the fruit evenly in the bin to prevent too much weight pressing on it. The procedure of holding the fruit in storage bins in order to reduce juice acidity and increase the final juice ratio needs to be applied with care because passing fruit through bins usually reduces the final juice yield compared to direct processing.
Besides the main function of storing fruit with different characteristics to give processing options and yield the desired juice quality, correct management of stored fruit also makes it possible to avoid wide fluctuations in the Brix/acid ratio of raw juice. Such fluctuations are usually the main cause of hesperidin-related defects formed during evaporator operation and found in the final juice concentrate. A good procedure is to minimize the time fruit stays in storage bins, preferably to less than 24 hours. Storage for longer times, however, does occur.
Surge bin
Fruit is drawn from the storage bins into the surge bin, where fruit from one or more storage bins may be combined.
Final fruit washing
The fruit is thoroughly washed immediately prior to the extraction process. The wash water may include a mild disinfectant to help reduce microbial presence on the fruit surface. Fresh water or condensate recovered from the evaporators is used for final washing.
Final grading
The fruit passes over a series of grading tables for final visual inspection where damaged or unsuitable fruit is removed.
5.4 Juice extraction
The aim of the juice extraction process (see Figure 5.4) is to obtain as much juice from the fruit as possible while preventing rag, oil and other fruit components from entering the juice. These may lead to bitterness in taste or other defects during subsequent juice storage.
The extraction operation determines product quality and yield and thus has a major effect on the total economics of the fruit processing operation. Once the fruit has been washed and graded (inspected), it is ready for the extraction process. To optimize extractor performance, the raw fruit must be sorted according to size because individual extractors are set to handle fruit of only a certain size range.
Fruit sizing
After grading, the fruit passes over the sizing table, which divides the fruit into different streams according to fruit diameter. A sizing table is generally made up of a series of rotating rollers over which the fruit passes. The distance between the rollers is preset, and increases as the fruit travels over the table. Over the first set of rollers, the smallest fruit drop between the gap onto a conveyor that carries them to an extractor set for their particular size range.
As the gap increases, larger fruit will pass through the rollers onto extractors set for their defined size range. In this way, all the fruit is selected to suit the individual settings of the extractors. There are normally two to three different size settings in an extractor line.
A fully functional fruit sizer is essential for producing juice of high quality and/or yield. If the fruit is too big or small, then (depending on the extractor type) it will be over-squeezed and excessive rag and peel will get into the juice with resulting bitterness. If the fruit is under-squeezed, insufficient yield will result.
Oil emulsion, containing oil from the peel and water, goes to peel oil recovery.
Wet peel, along with pulp, rag and seeds, flows directly to the feed mill.
Pulpy juice goes to clarification and then production of concentrate or NFC. Pulp intended for sale as pulp goes to pulp production. Residual pulp goes to pulp washing or the feed mill.
5.4.1 Extractor types
Two types of extractors dominate in orange processing plants, the squeezer type and the reamer type. For these two types there are two major brands: JBT (formerly FMC) for squeezer type and Brown for reamer type. Both extraction systems are dedicated to citrus fruit.
The reamer-type extraction system provides excellent separation of the orange components juice, oil and peel.
The process works best, as regards both product and yield, with fruit round in shape and of uniform ripeness such as found with Florida fruit. Squeezer-type extractors are less sensitive to fruit size and shape but can lead to higher oil content in the juice and a higher ratio of damaged pulp than reamer-type extractors. Adjustments to the standard squeezer-type extractor may be needed to keep oil levels low and/or improve pulp quality.
Squeezer-type extractors are the most common at global level. In Florida, however, the installed extraction capacity is about equal for both types of extractors. A majority of the NFC produced in Florida is extracted using reamer-type extractors.
The rotary press extractor is another type of extraction equipment – a multi-purpose machine that can also be used to process other types of fruit.
A rotary press extractor slices the fruit in half and passes the halves between rotating cylinders that press out the juice. Oil is extracted from the peel in a separate step prior to extraction. Although the extraction process is simple, both juice yield and quality are less optimal compared to squeezer-type and reamer-type extractors.
Rotary press extractors, which have high per-unit capacity and require lower investment, are popular in the Mediterranean region. Globally, however, they are of minor importance compared to squeezer- and reamer-type extractors.
Once installed in a plant, extraction systems are not easily interchangeable due to the different demands on the surrounding equipment.
5.4.2 The squeezer-type extractor
Figure 5.5 shows a squeezer-type extractor. This equipment is placed in lines in the extractor room, with up to 15 extractors per line. Each extractor may be fitted with five heads, which are available in different sizes so that they can handle the type and quality of fruit available. Typical sizes are 2 3⁄8, 3, 4 and even 5 inches (used mainly for grapefruit). The head size for each extractor in a line is chosen to optimize the handling of sized fruit. The extractor separates the fruit into four parts – pulpy juice, peel, core (rag, seeds and pulp) and oil emulsion.
The head of an extractor comprises an upper and a lower cup (see Figure 5.6). The cups have metal fingers that mesh together as the upper cup is lowered onto the lower cup. A cutter comes up through the centre of the lower cup to cut a hole through the skin in order to allow the inner parts of the orange to flow out. The cutter is part of the perforated strainer tube, sometimes referred to as the pre-finisher.
Once the strainer tube has cut into the fruit, the upper cup squeezes down on the lower cup. This pressure initially forces the juice to burst out of the juice vesicles and pass through the perforations of the strainer tube. Remnants of the ruptured juice sacs will pass through with the juice as pulp. The upper cup continues to squeeze down on the lower cup to extract as much juice as possible.
Eventually, the downward pressure causes the peel to break up, disintegrate and pass up through the fingers of each cup. Juice flows through the strainer tube into the juice manifold. The core material is discharged from the bottom of the strainer tube through the orifice tube.
As the peel is forced through the fingers in the cup during the last step of the extraction cycle, oil is released from the peel. The fragments of peel are washed with recycled water to extract the oil from the oil sacs. The oil is discharged from the extractors as an emulsion with water.
The squeezer-type extractor separates the fruit into four principal product streams in one basic step. It is claimed that contact is avoided between the juice and oil, and the juice and peel.
For successful operation of this equipment, correct cup size selection and adjustment of cup and cutter operation are paramount. When undersize cups are used, excess pressure is applied to the fruit, and the fruit may be blown out and/or peel may enter the juice stream. If too little pressure is applied, the yield will drop.
The throughput of a five-head extractor will vary according to fruit quality and size. The standard operating speed is 100 rpm, or 500 oranges per minute. Fruit will not always flow to each cup: 90% utilization is a high figure. A typical capacity for medium-sized fruit is 5 tonnes/h of fruit per extractor, corresponding to about 2,500 l/h of juice.
Because extractors operate at a fixed number of oranges processed per minute, the citrus processing plant throughput is very sensitive and dependent on fruit size. Processing small fruit (350 oranges/box) will result in 1,500 l/h juice flow rate instead of 3,000 l/h when processing large fruit (180 oranges/box). This difference means a doubling of juice volume and by-products to be handled by downstream equipment. These figures show the importance of correct storage bin management and fruit size in smooth factory operations.
There are two major types of extractor system, squeezer type and reamer type
Modifications for premium pulp
As the pulpy juice passes through the holes in the strainer tube in the standard extractor, the pulp tends to be broken up into small pieces, typically 2-3 mm in length or less. This is acceptable if the pulp is intended for pulp wash and as commercial pulp for certain markets.
Market demands in the juice market are changing, and the need for more “natural” pulp that has been subjected to less shear is increasing. In a squeezer-type extractor of modified design, larger pulp pieces of up to 15-20 mm in length flow with the juice stream. The main difference in design is the use of a modified strainer tube with larger openings that allow more pulp to remain in the juice stream. The pulp is subsequently separated from the juice and treated in a modified pulp recovery system.
Modifications to the squeezer-type extractor to produce premium pulp were introduced in the 1990s. There are several installations in Florida and Brazil.
Premium juice “low-oil” extractor
Certain fruit varieties, such as the Florida Valencia, will express more oil into the juice stream than other varieties. This can lead to oil content in the juice exceeding acceptable levels (such as 0.035%, the maximum level permitted in Florida for grade A juice).
This is a problem with NFC but less so with juice intended for concentrate because most of the oil will flash off in the evaporator. In the low-oil version of the squeezer-type extractor, the design of the strainer tube and orifice tube area are modified. This unit cuts a smaller core and puts less pressure on the fruit during extraction, thereby reducing the amount of peel oil that enters the juice. These modifications may also lead to a reduction in juice yield. Stopping the top spray of water reduces the amount of peel oil to be recovered.
Alternatively, hermetic centrifuges or vacuum flashing can be used in conjunction with standard extractors to deoil the single-strength juice. This allows a higher juice yield to be maintained during extraction, while excess oil is removed after the extraction process.
5.4.3 The reamer-type extractor
The reamer-type extractor is based on the same principle as a typical manual kitchen squeezer used for making orange juice at breakfast. An extraction line comprises several extractors, and it is very important to set up each extractor to suit the size of fruit fed into it. A reamer-type extractor is illustrated in Figure 5.7.
Fruit is fed into the feed wheel and cut in half. The halves are oriented and picked up in synthetic rubber cups mounted on a continuous belt system. A series of nylon reamers (cone-shaped inserts that have ridges moulded into the form from tip to base of the reamer) are mounted on a rotating turntable.
The reamers, aligned in the vertical plane on most models, enter each fruit half and rotate as they penetrate them. The speed of rotation varies as the reamer penetrates the fruit, being slower towards the end of the operation. Juice, pulp, rag and seeds pass out through one outlet, and the remaining peel passes out through the peel chute.
A strainer separates the juice and pulp from the rag and seeds and the flow then passes to the finishers. The size, pressure and rotation speed of the reamer can be adjusted to suit fruit maturity, size and quality.
The reamer-type system typically gives higher-quality pulp (longer and larger cell fragments) than standard squeezer type extractors. Juice yields between the two systems are comparable.
The oil extraction system
Peel oil can be recovered from orange peel using a separate oil extraction system that is placed upstream of the juice extractors. It operates on the principle of puncturing oil sacs in the flavedo and washing the oil out to make an emulsion (see Figure 5.8). In the first stage of the oil extraction system, whole fruit passes over a series of rollers with small but sharp needle-like projections. The oil glands are pricked rather than scraped open so that little damage is done to the peel. Hence, the amount of non-oil material washed away with the oil is minimal. This, in turn, makes the water stream separated from the emulsion cleaner and easier to recycle.
The rollers conveying fruit are placed in a water bath and the oil from the pierced glands is washed out with water. After a finishing (straining) stage to remove any large particles of peel, the oil-water emulsion can be concentrated and polished in a series of centrifuges (see subsection 5.8 on peel oil recovery). The water is largely recycled.
The reamer-type extraction system requires two separate steps to extract juice and oil from the fruit. Nevertheless, the oil emulsion is often considered cleaner, easier to centrifuge compared to other types of oil recovery system, and the extracted juice has less contact with the oil.
5.4.4 Downstream of the juice extractors
The juice streams from a squeezer-type extractor line or reamer-type extractor system flow to clarification and then evaporation, or pasteurization if the end-product is NFC. The oil emulsion flows to peel oil recovery for separation by centrifugation. Peel, rag, seeds and other solid material are conveyed to the feed mill.
5.5 Clarification
The juice leaving the extraction process is clarified as it contains too much pulp and membrane material to be processed in the evaporator or as NFC. Figure 5.9 shows the typical process steps for juice clarification.
Pulpy juice from the extractors generally contains around 20-25% of floating and sinking pulp. Clarification involves finishing the juice that is removing the pulp. A finisher is basically a cylindrical sieving screen and comes in one of two types: screw-type and paddle. Figures 5.10 and 5.11 illustrate how they work.
Screw-type finishers
Screw-type finishers have a stainless-steel screw that conveys the pulpy juice through the unit and presses the pulp against the cylindrical screen. The juice flows through the screen holes.
The pulp is thus “concentrated” inside the screen and is discharged at the end of the finisher. As pulp is discharged through a restricted opening, the resulting back-pressure in the finisher helps to squeeze out more juice from the pulp mass.
Paddle finishers
Paddle finishers incorporate a set of paddles rotating on a central shaft within the cylinder. The paddles push the pulp against the screen, applying centrifugal force rather than pressure to separate the pulp from the juice. This usually provides gentler pulp treatment and higher flow rates than screw finishers.
Two finishers are often placed in series at the end of the extraction line. The upstream primary finisher is not set as tightly as the downstream secondary unit and thus will have a higher flow capacity.
The exact configuration of the clarification stage depends on the manufacturer of the extractor system and the type of pulp that the processor intends to recover. The pulpy juice stream from a reamer-type system or premium pulp squeezer-type extractor may first pass through a classifying finisher (with larger holes) to remove peel and membrane pieces before pulp recovery.
The standard squeezer-type extractor includes a pre-finishing tube in the extractor and the pulpy juice flows directly to the primary finisher.
Centrifugal clarification
The pulp content of juice leaving the secondary finisher is typically about 12%. This pulp is predominately sinking pulp. The juice may also contain seed fragments (black specs), filaments of core material and other undesirable high-density material. Centrifugal equipment is used to remove these defects from the juice. Clarifying centrifuges are also employed to reduce pulp content to the level specified by the market for final juice.
A two-phase clarifier separates the juice into a liquid phase and a solid phase. Separation in the disc stack centrifuge takes place in the spaces created between a number of conical discs that are stacked on top of each other to provide a large separation area. Most models rotate at between 4,000 and 10,000 rpm. The accumulated solids can be discharged – without having to stop the centrifuge – by rapidly opening an annular slot at the periphery of the rotating bowl. The clarified juice leaves the centrifuge under pressure. Clarification by centrifugation often leads to improved operation of the evaporator system by providing consistent juice pulp levels.
Producers of orange drinks and nectars frequently specify FCOJ with “very low” or “low” pulp content, which refers to <1% and <3% sinking pulp respectively (measured in reconstituted juice). To achieve such low levels in a traditional centrifuge, pulp discharge intervals need to be very short, which results in flow variations and high equipment wear. Alternatively, a new type of hermetic depulping centrifuge can be used to overcome these issues while achieving the desired quality. The three-phase depulping centrifuge ejects low-pulp juice and concentrated pulp continuously, which enables the separation of large quantities of high-concentration pulp. Only the solid phase, containing black specs, is discharged intermittently. Usually, this type of machine is situated in the evaporator system, between stages, so that it treats warm juice. The centrifuge controls juice pulp content as well as the dryness of the ejected pulp. This eliminates the need for a juice recirculation system.
Turbofilters
Turbofilters were introduced in Brazil during the mid 1990s as an alternative to screw and paddle finishers. Turbofilters are claimed to give a more stable level of sinking pulp in the finished juice than conventional finishers. They incorporate a stainless-steel conveyor, which rotates faster than screw finishers and pushes the pulpy juice against a plastic screen. The pulp content of the juice can be adjusted by changing the inclination of the turbofilter.
Blending
After clarification, the juice often undergoes some degree of blending with juice from other batches to balance its flavour, colour, acidity and °Brix levels before further processing. If intended for NFC production, the juice leaving the clarification section should be cooled to 4°C to minimize potential microbiological activity before being passed into the buffer/blending tanks.
5.6 NFC production
The aim of NFC processing is to produce orange juice using the minimum of thermal processing. Nevertheless, the thermal treatment should be sufficient to ensure that the product is physically and microbiologically stable. Because fruit harvesting is seasonal and juice consumption is year-round, the product must be stable enough to be stored for several months up to one year so that seasons can be bridged.
In some instances, NFC is pasteurized and packaged for the retail market during the season without long term bulk storage. When this is the case, some blending may occur following the clarification step to minimize hourly variations in acidity and °Brix. Some pulp may also be added depending on market demands.
More commonly, the juice is processed and stored in bulk under aseptic or frozen conditions for some months until it is reprocessed and packaged. For large-volume NFC production, as in Florida and Brazil, aseptic tank farms are the most common form of NFC storage.
5.6.1 Oil reduction
Oil content in the juice from extraction may exceed acceptable amounts, depending on fruit variety and extractor operation. The levels may be dictated by regulatory standards. For example, Florida grade A juice may have a maximum oil content of 0.035%. Alternatively, oil content may be decided on the basis of consumer preference. Acceptable levels of oil in ready-for-consumption juice range from 0.015 to 0.030%.
Oil content can be reduced in different ways:
Adjusting the extractor
Less pressure is applied to fruit during extraction or a low-oil extractor (squeezer-type) is used. Both alternatives are likely to reduce juice yield.
Vacuum flashing of preheated juice
This method is based on the vapour pressure equilibrium between water, d-limonene and volatile flavour compounds. When the pre-heated juice enters the vacuum chamber it starts to boil, evaporating low boiling point compounds, oil and 1-2% water. The vapours are condensed in an external plate heat exchanger and the chilled condensate flows to a decanter vessel. Here the water phase essence is separated from oil by gravity and returned back to the main juice stream. The oil phase (d-limonene) is collected in a different stream for other uses.
Centrifugal separation of the oil phase from the clarified juice
This method allows juice yield from the extractors to be maintained at a high level and there is no heating of the juice.
Deoiling with centrifuges
Removal of oil from single-strength juice with centrifuges has been practised for years. It is a difficult separation task because the oil droplets are well emulsified, particularly in juice from squeezer-type extractors. Hermetic centrifuges give good results in separating oil even from juice processed by squeezer-type extractors.
In a hermetic centrifuge the rotating bowl is completely filled with liquid. This avoids air pockets and any free liquid surfaces in the bowl, which in turn avoids air entrainment and high shear forces.
The feed enters the centrifuge bowl from underneath through a hollow spindle (see Figure 5.13). The smooth acceleration of the product as it enters the centrifuge prevents scattering of the oil globules, thereby enhancing separation. The hermetic (gas-tight) design also prevents loss of volatile components in the juice and oxygen ingress.
Deoiling single-strength juice with hermetic centrifuges can typically reduce oil concentrations from 0.04- 0.08% to 0.02-0.035%. In terms of juice yield, the use of a deoiling centrifuge in combination with standard extractors gives a yield increase of 2-4% compared to an extractor fitted with low-oil components.
The deoiled juice is buffer-stored for a short period prior to pasteurization. Some blending to balance quality variations may be carried out.
5.6.2 Primary pasteurization
Pasteurization prior to storage – the primary pasteurization – must achieve two goals. It must deactivate the enzymes present in the juice and make the juice microbiologically stable (see subsection 4.4). It is carried out using tubular or plate heat exchangers. The choice of heat exchanger depends on the amount and type of pulp in the product and on the processor’s preference. Tubular heat exchangers are best for juice containing floating pulp (see subsection 7.5). After bulk storage the juice is usually pasteurized at least a second time prior to filling in retail packages.
The long shelf life required for NFC destined for bulk storage demands strict attention to hygiene. Single-strength juice is more sensitive to microbial contamination than concentrate (where the high osmotic pressure resulting from high sugar content retards microbial growth). The use of chilled storage instead of frozen storage also imposes much stricter hygiene requirements for NFC production than what FCOJ producers may be accustomed to.
Good manufacturing practice demands that the pasteurizer system is pre-sterilized at 95°C or higher prior to production and that a CIP programme is integrated with the control system. NFC volumes for processing are normally large, so a high degree of energy recovery is advisable.
Dissolved oxygen in juice causes loss of vitamin C during storage
Thermal treatment is a concern among many NFC producers. Excessive heat load on the juice should be avoided. Careful control of temperature and residence time using well-designed heat exchangers is important. Low temperature differentials between the heating medium (hot water) and product minimizes “shock” to the product.
The purpose of primary pasteurization is two-fold
- To deactivate enzymes
- To make the juice microbiologically stable
5.6.3 Deaeration
Air tends to get mixed into the juice in the extractors and finishers. Some of the entrained air may escape during buffer storage, but juice going to pasteurization is normally saturated with dissolved oxygen. It also contains some free air. During product storage, dissolved and free-bubble oxygen in the juice may destroy a significant amount of the available vitamin C by oxidation (see section 4). Air bubbles present in the product during pasteurization may also lead to insufficient heat treatment.
Inclusion of deaeration as part of the pasteurization process is therefore recommended when producing NFC. Deaeration is usually carried out by passing the product through a vacuum chamber. Free air bubbles expand in a vacuum and tend to escape quite easily from the juice, though dissolved oxygen is more difficult to remove.
The deaeration efficiency, or reduction of dissolved oxygen, depends on several operating factors including the vacuum applied and juice surface area in the deaerator. Deaerator operation and equipment are described in subsection 7.5.
Volatiles that flash off during deaeration are condensed and returned to the juice stream. Alternatively, they are sometimes removed and stored separately from the bulk juice.
5.6.4 Long-term frozen storage
After primary pasteurization, orange juice is stored in bulk under either frozen or aseptic conditions. NFC production involves large product volumes. For the same amount of final juice, NFC volumes are five to six times higher than FCOJ.
Freezing minimizes vitamin degradation and changes in flavour during the storage period, but the energy and warehouse costs of freezing and storing frozen NFC are high.
Freezing of NFC leads to handling problems because it freezes solid, whereas frozen orange concentrate is very viscous but still pumpable. Frozen NFC storage is more appropriate to low NFC volumes. High-volume producers store NFC aseptically in very large tanks.
Juice to be stored frozen is filled in mild steel 200 litre (55 US gallon) drums lined with a polyethylene plastic bag. As the product is to be frozen, the net filling volume is about 170 litre (45 US gallon).
Alternatively, the juice can be poured into block formers and then frozen (mainly for on-site storage). The frozen product is usually kept at -18°C or lower.
Thawing of NFC to make it ready for final processing also leads to some logistical and handling difficulties. It takes several days or weeks for bulk product in drums to thaw at ambient temperature. The outer layer of juice may be exposed to microbiological contamination during thawing, with a negative impact on product quality. Crushing systems enable more rapid handling but require higher energy consumption and capital investment.
Systems for freezing larger blocks of juice incorporating novel techniques for rapid freezing and thawing have been introduced, but so far have limited commercial use.
There are three major options for long-term storage of NFC juice
- Frozen storage
- Aseptic storage in tanks
- Aseptic storage in bag-in-box bulk containers
5.6.5 Aseptic storage in tanks
As an alternative to frozen storage, NFC may be stored chilled in aseptic tanks. Technology exists to build very large tanks with capacity of up to 6 million litres for aseptic juice storage. Unique production techniques are employed to coat the internal surfaces of the carbon steel tanks with an epoxy lining. While lined tanks are common in Florida, Brazilian producers use mainly stainless-steel tanks for NFC storage.
The tanks are sterilized prior to filling by flooding them with a sterilizing fluid such as iodoform. To reduce water consumption, Brazilian processors are also adopting an alternative sterilization method where the sterilant (peracetic acid) is injected as a fine mist into the tank. The micro-sized particles reach the stainless steel surfaces and provide the desired sterilization effect usually after 24 hours contact time.
The preferred storage temperature is about -1°C, just above the juice’s freezing temperature. Instead of being fitted with cooling jackets, the tanks are housed in a large refrigerated building.
The juice must be agitated periodically to avoid separation of sinking pulp and to maintain °Brix uniformity. A pressurized nitrogen blanket is often maintained above the juice surface to minimize the risk of vitamin C loss through oxidation.
When product is required from the tanks, it is typically drawn off, blended with juice from another time in the season (and perhaps pulp) and repasteurized.
The technology to operate very large aseptic tanks was pioneered in Florida and gave rise to rapid growth in storage capacity during the 1990s and early 2000s. Growth in NFC production in Brazil after 2000 has also led to the construction of large storage tanks. In Florida, the NFC is mainly stored and utilized on a processor’s site. NFC produced in Brazil, however, is primarily intended for export. The first aseptic tank farms were therefore installed dockside at the export terminal. Port space restrictions mean that additional aseptic storage required to meet increased NFC production is now built at the juice plants.
Clarified juice intended for storage at the export terminal is pre-pasteurized and cooled to 0°C at the processing site. It is then transported by road tanker to the port. (The distance from one of the major citrus processing sites to Port of Santos is 450 km.) Full juice pasteurization takes place at the terminal before the juice is transferred to the large aseptic storage tanks for long-term storage to bridge the seasons.
Technology has been developed to avoid repasteurization prior to export. The technology allows the aseptic transfer of juice from on-land storage to sea vessels fitted with bulk aseptic tanks. Juice is transported to the US and Europe under chilled aseptic conditions on specially designed ships (see subsection 6.3.3). On arrival at its destination, the juice is aseptically transferred to aseptic dockside tanks at the reception terminal using similar technology to that at the export facilities.
Large aseptic tank storage facilities now exist in other NFC-producing regions, for instance in Spain. Road tankers transport chilled NFC year-round in bulk from Spanish fruit processors to juice packers across Europe.
Aseptic NFC technology requires a substantial upfront investment, and the value of product at risk when stored in such large tanks is considerable. Today, however, risks are minimized by field-proven designs, advanced aseptic technology and more than 20 years’ experience of the many large tank systems installed.
As an alternative to frozen storage, NFC may be stored chilled in large aseptic tanks
5.6.6 Aseptic storage in bag-in-box bulk containers
As an alternative to aseptic tanks, the juice may be filled in 1,000 litre (300 gallon) aseptic bag-in-box containers (see Figure 5.14). The bags, placed in bins usually made from wood, are then stored under refrigerated conditions. After storage, the product is accessed by opening the bag and pumping out the product. Alternatively, the bag can be emptied and the juice transferred aseptically to the filler.
The use of “one tonne” aseptic bag-in-box containers for NFC storage requires more labour for filling and emptying the containers compared to large tanks. However, it gives added flexibility regarding storage capacity due to the moderate level of investment required to store additional juice volumes. A drawback of the aseptic tank approach is finite storage volume in the absence of a major investment in reserve capacity. Consequently, the bag-in-box solution is often preferred for start-up operations for NFC production. NFC processors who already have aseptic tanks installed may also use bag-in-box containers to provide additional storage capacity and shipment of NFC.
Aseptic security during product filling and storage in the bag must be high. Any contamination may lead to blown bags during storage and shipment. Needless to say, a single blown bag during shipment can cause significant trouble.
For long-term juice storage (six months or more), bag material with a very effective oxygen barrier is recommended. Bags made with foil-based aluminium laminate offer higher protection against oxygen than metallized laminates in which the aluminium layer is much thinner.
Several filling systems are in operation for aseptic bag-in-box (BiB) containers. Some evolved from conventional (non-aseptic) BiB systems. A sterile chamber surrounds the filling head and chemical sterilants are used for sterilization.
Other systems were developed specifically for aseptic filling, an example of which is shown in Figure 5.14. It incorporates a simple filling system (spout and filling valve) and uses steam as the sterilizing agent.
5.6.7 NFC reprocessing
In the US, some NFC is moved in bulk by road and rail tanker to juice packers across the country, but most NFC is filled in retail packages in Florida and distributed from there. Shipping from Florida to Europe in bulk is done in frozen drums and aseptic bag-in-box containers. For Brazilian NFC, overseas shipping also occurs by bulk sea tanker. Overseas shipping of packaged product is at a cost disadvantage compared to shipping bulk juice. Moreover, the additional delay in transport time adds to difficulties with logistics and forecasting for the packaged product.
Traditionally, NFC taken from storage at the fruit processor’s site to be reprocessed is blended with juice from a different time in the season and/or with pulp. The juice blend is then repasteurized prior to filling in consumer packages.
Alternatively, specially designed equipment can be used to transfer juice from aseptic bulk bags to consumer packages via an aseptic tank, without the need for repasteurization.
When NFC is shipped overseas, blending with other orange juice varieties and pulp is carried out at the reception terminal’s juice facilities or at a blending house.
5.7 Concentrate production
Globally, the majority of orange juice is produced as concentrate. Juice from the clarification step is evaporated to remove most of the water (see Figure 5.15). Currently, the citrus evaporators in widest use are of tubular design, though some citrus plants use plate and cassette evaporators.
Evaporators are designed to handle the huge juice volumes commonly processed in large citrus plants. Evaporator capacities can exceed 100,000 kg/h (about 220,000 lbs/h) of water evaporated. Versions with as many as seven effects are in operation (seven effects means essentially that the steam is reused to evaporate water in seven steps). Such systems have a very low specific steam consumption: only 1 kg (2.2 lbs) of steam is used to evaporate 6 kg (13.2 lbs) of water. However, additional effects increase the residence time of the product in the evaporator.
The 1970s and 1980s saw a large expansion of concentrate capacity in the major citrus markets of Brazil and Florida. Today, little increase in evaporator capacity is needed in these regions, but new evaporators are being installed to satisfy the requirements of other expanding orange-producing regions.
5.7.1 Tubular evaporator systems
The most common type of tubular evaporator system used for orange juice is the thermally accelerated short-time evaporator (TASTE). It is generally described as a continuous, high-temperature short-time evaporator of the long, vertical tube falling-film type. It was designed and developed in Florida but is today manufactured in many different countries. These evaporator systems are dedicated to citrus fruit.
Figure 5.16 shows a flow diagram of an evaporator with seven product stages. The juice is first preheated to 95-98°C. Holding at pasteurization temperature stabilizes the juice by means of microbial and enzyme inactivation. The product then passes through a number of stages under vacuum until a concentration of up to 66 °Brix is achieved. By this time the product temperature has fallen to about 40°C. The residence time in the evaporator is typically 5-7 minutes or longer.
Good distribution is of primary importance in evaporator design. It ensures that all the product is uniformly treated and that the heat exchange surface is used to its maximum potential.
A special feature of the TASTE evaporator is the way in which the product is distributed across the tube bundle. The juice is fed into the distribution section at a temperature and pressure greater than in the entry zone of the tube bundle. It enters through a diverging expansion nozzle that converts all of the product into a liquid/vapour mixture. The expanding vapour accelerates the liquid/vapour mixture through a second nozzle and cone assembly. Further flash expansion of the vapour causes atomization of the liquid phase into a turbulent mist. The acceleration effect can cause mist velocities to exceed 50 metres/second on leaving the tube bundle! The high degree of turbulence increases heat-transfer rates and reduces burn-on, which helps to achieve long operating runs.
Other tubular evaporation systems
There are also other tubular evaporator systems of similar design for citrus plants, including a conventional mechanical method of distributing product across the tube bundles. They incorporate thermal recompression to increase steam economy without increasing residence time. Relatively few of these evaporator systems have been installed for high product capacities.
5.7.2 Plate and cassette evaporator systems
In addition to tubular systems, plate and cassette evaporators are also used to concentrate orange juice. As the name implies, plate evaporators consist of plates clamped together in a frame with gaskets between them. It is more common to use cassettes (welded double plates) instead of single plates because they simplify maintenance. The heating medium (steam or vapour) flows through the space between the welded plates that make up the cassette.
Product channels are formed between individual cassettes separated by gaskets. This configuration allows ready inspection of product surfaces.
Some advantages of plate/cassette evaporators compared to other types are easy capacity increases by adding more cassettes, and simplified maintenance and inspection by opening the frame. Also, the compactness of cassette evaporators eliminates the need for several access walkways on different levels, as are required for tubular systems. The one-level configuration offers easy operator control and ready access for maintenance activities.
A small temperature difference between the product and heating medium is sufficient in this type of evaporator. This allows lower operating temperatures to be used than with traditional tubular evaporators. The main type of cassette evaporator is the rising film unit.
Rising film cassette evaporator
In a rising film cassette evaporator, the product enters the bottom of the cassette and rises over the heating surface as it boils (Figure 5.17). No mechanical feed distribution device is needed and even distribution is achieved through gravity.
The film flows upwards in a homogenous flow, thus giving a very short retention time over the heating surface. The liquid/vapour velocity is very high at the outlet of the short heating channel. It is possible to evaporate products of higher viscosity and higher pulp content than in a falling film evaporator.
As plate systems can be designed for flexibility, some installations installed in citrus plants are used to process other types of juice outside the orange juice season.
5.7.3 Homogenization
Homogenization of concentrate sometimes takes place in the evaporator system. Product then normally passes through a homogenizer before the seventh and last concentration step. At this stage the concentration is approximately 40-42 °Brix. Homogenization breaks down the pectin, thereby lowering the viscosity of the concentrate. This increases the efficiency of the final stage of the evaporator.
Another important benefit of homogenization is the disintegration of hesperidin flakes that may be present in the concentrate. Hesperidin is a flavonoid that forms white crystals in juice (and is part of the juice cloud). During evaporation the crystallization rate increases, resulting in large white flakes. These tend to build up a fouling film on the evaporator surfaces and may be released into the concentrate during production. It is known that changes in the Brix/acid ratio of the processed juices cause white flakes to loosen from the metal surfaces. This necessitates cleaning-in-place (CIP) of the evaporator. The presence of hesperidin flakes in concentrate is a defect and results in a lower USDA quality score.
It is also claimed that homogenization reduces sinking pulp level in the product. This could permit juice with higher pulp levels to be fed to the evaporator.
In the case of special requirements of concentrate with very low pulp content (<1%), specially designed hermetic clarifying centrifuges may be included in the evaporator system to reduce pulp to very low levels.
5.7.4 Essence recovery
During the evaporation process, volatile juice components are stripped from the juice along with water. These components are often recovered in an essence recovery system connected to the evaporator. The essence process usually forms an integral part of the mass and thermal balance of the evaporator system. Dr James Redd of Florida pioneered the design development of essence recovery units, with the first commercial system being installed in 1963.
The vapours from the early product stages of the evaporator contain most of the volatiles from the juice. These are captured and sent to a still mounted on the evaporator. The important volatiles are separated from the water by distillation under vacuum and condensed by chilling. The product essence is a concentrated mixture of aqueous and oil-soluble aroma compounds. This essence is separated into oil and aqueous phases either by decantation or centrifugation.
A more recent design of essence recovery systems uses structured packing in the distillation column instead of the traditional perforated plate device. This new approach results in less aroma liquid hold-up volume and residence time as well as increased separation efficiency. These features help to improve the yield of both water phase aroma components and essence oil.
Water phase aroma and essence oil
The aqueous phase (called water phase aroma or essence aroma) contains the flavour top-notes. It has an alcohol strength typically standardized at 12-15%. The oil phase (essence oil) holds the fruity and sweet-tasting flavours of fresh juice. Its flavour properties differ from those of peel oil (see section 8). Add-back of water phase aroma and essence oil to concentrate has replaced the previous practice of adding single-strength juice (called cut-back) to improve concentrate flavour.
In Florida, Valencia oranges, a late variety, are used to produce the best essence. By contrast, orange varieties harvested early in the season yield little essence, and it is often of poorer quality.
Aroma and essence oil are either sold as separate products to concentrate blending houses and juice packers or sold on contract to specialty flavour manufacturing companies.
5.7.5 Concentrate storage
After evaporation, the 65 °Brix concentrate is chilled to -10°C and then routed to storage. Blending of different production lots and addition of peel oil and essences may be done on the way to concentrate storage. Storage takes place in bulk storage tanks or 200 litre drums with plastic liner. Drum storage is normally maintained at -20 to -25°C, bulk storage in large tanks often at -10°C. Just prior to dispatch from the plant, concentrate drawn from different bulk storage tanks is often blended to meet product specifications. Concentrates are sometimes diluted with pulp, for example, to reduce the Brix level. Shipping of frozen concentrate involves drums, tank cars or bulk tanker ships.
Concentrate is traded as FCOJ. The term “frozen” may be misleading as concentrate at 65 °Brix does not freeze solid at -10°C due to its high sugar content. The most common FCOJ concentration is 65-66 °Brix, but bulk concentrates of lower °Brix are also available. FCOJ of 55-58 °Brix is typically supplied to dairies.
5.7.6 Alternative concentration methods
Alternatives to evaporation for concentrating orange juice have been developed and tested, but so far have enjoyed limited commercial application. Lower concentrate Brix levels and high operating costs compared to standard evaporator systems have hindered the commercialization of the new systems.
Two methods that do not use heat for concentration are freeze-concentration and membrane filtration.
Freeze-concentration
This method is based on the fact that water forms ice crystals during the freezing of sugar solutions. These can be separated from the solution, thereby increasing sugar concentration. It is necessary to inactivate enzymes when applying freeze-concentration to juice. Enzyme inactivation can be achieved by pasteurizing the juice before freezing or pasteurizing the resulting concentrate.
Several studies have shown that freeze-concentration yields superior flavour quality compared to conventional evaporation. However, the low temperatures involved result in high viscosities in the concentrated products. These limit both the degree of concentration that can be achieved and the amount of pulp and insoluble solids that may be present in the juice to be concentrated (Braddock and Sadler, 1989). Concentrates of up to 40 °Brix can be obtained with this method.
Membrane filtration
Membrane filtration is another evaluated method for concentrating orange juice without using heat. However, the high viscosity of the concentrate reduces filtration efficiency and limits the degree of concentration that can be achieved. To minimize viscosity, the pulp is first separated from the juice, for instance by ultrafiltration (UF), to leave a clear liquid (serum) that is concentrated by reverse osmosis.
The enzyme-rich pulpy stream is pasteurized before being recombined with the serum concentrate. Mixing back the insoluble solids stream, essentially at single-strength juice concentration, reduces the Brix value of the concentrate. Concentrations of up to 42 °Brix have been reported.
Concentration systems using other membrane processes have also been tested. However, the necessity of retaining the sugars, acids and aroma compounds to maintain a balanced citrus juice flavour puts heavy demands on potential membrane systems.
5.8 Peel oil (cold-pressed oil) recovery
The oil-water emulsion, or oil frit, from the extraction process is sent to the peel oil recovery section. Fruit substances – including peel and pulp particles and soluble pectin and sugars – are present in the emulsion along with oil and water. Peel oil recovery systems aim to recover pure oil by removing all other substances with as little oil loss as possible. Peel oil is commonly referred to as cold-pressed oil.
5.8.1 Straining and concentration step
The first step involves using a finisher as a straining method to remove large pieces of peel and other orange parts that need to be excluded from downstream centrifuges (see Figure 5.19). After straining, the oil emulsion – containing about 0.5-2.0% oil – enters the first stage centrifuge (also called a desludger or concentrator). The centrifuge concentrates the oil up to 70-90%.
The first centrifuge is a three-phase machine. The light phase is concentrated oil, the heavy phase is water, and the third phase is residual particulate matter. The control of solids discharge from the sludge space is critical to the overall performance of the oil recovery system. Product is lost if the discharge frequency is set too high. Conversely, separation efficiency is lost if the sludge space is allowed to fill up.
The water stream is often recycled back to the oil extraction system as spray water, though it is important that some water is removed from the system to allow additional fresh water to enter. Microbiological problems may occur if the same water is continuously recycled. Moreover, the centrifuged water contains undesirable components such as soluble pectin.
As the concentration of these components builds up in the emulsion, oil separation efficiency decreases and oil yield declines. Again, this limits the amount of water recycling possible.
The centrifuged water also contains microscopic oil globules that are too small to be separated by the centrifuge. As this level of oil builds up with water recycling, the effectiveness of the water to extract oil from the peel decreases. This will also lead to an overall drop in oil recovery efficiency.
The type of oil extraction used and the performance of the centrifuges will determine the amount of water that can be recycled. The cleaner the peel oil emulsion is, the higher the oil yield is from the peel oil recovery system and the higher the volume of recycled water will be. The oil extraction system upstream of the reamer-type juice extractor is said to give a purer oil emulsion than the one-step squeezer-type extraction system.
For oil recovery, a hermetic centrifuge has several advantages over open-bowl-type design. The fully flooded bowl in the hermetic machine ensures that oil does not come into contact with air. The precise control of the oil-water interface leads to higher separation efficiency.
Figure 5.20 shows a hermetic centrifuge for concentration of peel oil emulsion.
5.8.2 Polishing
The concentrated oil stream then passes to a second-stage centrifugation process (polishing). Here, the oil is further concentrated to >99% purity. The flow rates are extremely small (1-2%) compared to flow rates in the first stage or in juice clarification and deoiling of single-strength juice.
Having already undergone one centrifugation process, the product is virtually free from solid particles. For smaller capacities, a solid bowl machine is used from which water and oil are continuously discharged. Periodic takedown removes any solid material that collects in the bowl periphery. For larger flow rates, a solids-ejecting polisher is used in which water and oil leave the machine under pressure. Accumulated solids are discharged about once or twice per hour.
One tonne of fruit typically yields 200-300 litres of emulsion to the first centrifuge and 3-6 litres of concentrated oil to the polisher.
Three-phase depulping centrifuges, which continuously discharge the light and heavy phases and intermittently eject solids, also have potential uses in cold-pressed oil recovery systems. They can increase oil yields thanks to their ability to remove pectins and insoluble solids from the “yellow water” stream. Depulping centrifuges were originally developed for efficient pulp removal in the production of low-pulp juices (see section 5.5).
5.8.3 The winterization process
The polished oil contains trace amounts of dissolved wax derived from the peel of the fruit. At temperatures above 15 or 20 °C, the wax is totally dissolved. However, at lower temperatures it may give a haze to the product. To avoid this problem the polished oil is dewaxed, or “winterized” as it is called.
The winterization process involves precipitating the wax by causing it to crystallize and then settle. The oil is stored in tanks at 1°C or lower, which causes the waxes to precipitate and sediment. The process typically takes 30 days or more, though at lower temperatures this period may be considerably shorter. The winterized oil is then decanted from the tank. Larger processors collect the sludge from different winterizing tanks so that once sufficient material has accumulated the waxes can be removed by centrifugation to recover residual oil.
The winterized oil is packed in 200 litre (55 US gallon) drums or road tankers. Normally the oil is stored under refrigeration (-10°C) and is traded as cold-pressed oil (CPO). Sometimes it is called cold-pressed peel oil (CPPO). It is used as a raw material in the flavour manufacturing industry and by concentrate blending houses and drinkbase manufacturers.
5.8.4 D-limonene recovery systems
The heavy phase from the peel oil first-stage centrifuge is usually recirculated to extractors as “yellow water”. Part of this flow (10-50%) needs to be replaced with fresh water to avoid excess concentrations of insoluble substances like pectin before this poor oil emulsion is sent back to the juice extractors.
The non-recirculated fraction of the yellow water has some residual oil content (0.5-1.0%) that instead of being sent to the feed mill or wastewater treatment is separated using equipment dedicated to recovering the residual oil. A traditional d-limonene recovery system heats the yellow water and further vaporizes a mixture of water vapour and d-limonene in one or more in-series flash chambers. The vapour is subsequently condensed to recover the terpenic fraction.
A more efficient new design eliminates the individual flash stages by incorporating a specially designed fractionation column to perform the vapour enrichment. This results in better d-limonene yields and quality and a more compact recovery unit. Both systems can usually be fed with sludge discharge from the first-stage centrifuge as well as from the polisher (second-stage) centrifuge in the peel oil recovery unit.
5.9 Feed mill operations
About 50% of the fruit remains after juice extraction. Much of this residual fruit matter is low-grade material in the form of peel, rag, core, seeds and pulp not used for commercial purposes.
Most larger processing plants have a feed mill that further processes this waste and recovers by-products.
Feed mill operations (see Figure 5.21) represent a significant part of total plant running costs. Solids drying and liquid stream evaporation are energy-intensive. Less waste and increased liquids recycling in other parts of the plant are desirable both for financial and environmental reasons. Regulatory pressure for environmental control in citrus plants continues to increase.
Revenue from the sale of feed mill by-products makes a significant contribution to orange processors’ overall profitability. Efforts are constantly being made to identify additional products that can be recovered from peel and other waste streams.
Feed mill process steps
The feed mill receives rejected fruit from the grading tables in the reception area and waste material from juice processing. This combined material, with moisture content of about 80%, is taken by screw conveyors to the wet peel bins of the feed mill. Hammer mills then break it down into small pieces. Small amounts of lime (0.15-0.25%) are added after this step to aid the dewatering process. After a dwell time of 10-15 minutes the mixture is conveyed or pumped to the peel presses.
The primary peel presses remove some 10% of the moisture. Continuous screw presses have largely replaced hydraulic batch presses for this task. Further addition of lime and secondary pressing can remove 2-3% extra moisture.
The liquid from the presses – press liquor – contains approximately 9-15% soluble solids, much of which are sugar solids. The oil content can be 0.2-0.8%. The press liquor normally flows over static screens to remove peel solids and then on to the waste heat evaporator.
Press liquor is usually concentrated to 50 °Brix and added back to the peel residue prior to pressing. Alternatively, it can be concentrated up to 72 °Brix and used as raw material for a fermentation process to make citrus alcohol.
Press liquor contains a high concentration of suspended materials and often includes sand-like matter. Decanter centrifuges that are used to clarify press liquor should be equipped with special internal tiles to minimize erosion. Clarification of the press liquor can prolong the running time of the waste heat evaporator and reduce cleaning time substantially, thereby enhancing feed mill cost-efficiency. The waste heater strips off d-limonene, which can be recovered as a separate stream from the vapour phase.
The pressed peel is dried in a rotary drier to a moisture content of about 10% and then pelletized to make animal feed. Vapour from the peel drier is used as a heating medium in the waste heat evaporator.
5.10 Pulp production
Floating pulp – larger solid particles in the juice – consists mainly of ruptured cell sacs and segment walls. It is separated from the juice in finishers. (The very small pulp particles flow with the juice stream from the finisher. These fine particles tend to sediment at the bottom of the juice and are referred to as sinking pulp.)
The pulp stream from the finisher is handled in different ways depending on the end use of the pulp. The alternatives are:
- Recovery for production of commercial pulp. Pulp is used as add-back in juice and juice drinks.
- Production of pulp wash – the juice sugars obtained by washing pulp with water. The remaining material is sold as “washed pulp” or taken to the feed mill.
- Routing to the feed mill for drying into pellets for animal feed.
In the past, most pulp went to pulp washing and the feed mill. However, since the market trend is to add more pulp cells to the final juice, a higher proportion of pulp from the extractors is going to commercial pulp production. Most processors, however, obtain more pulp from the fruit than is required by the juice industry for add-back to juice or in juice drinks. The extractor type and operation will influence the quality of the pulp produced. In some plants, the extractors used for pulp production are adjusted to optimize pulp quality rather than to maximize juice yield.
Figure 5.22 illustrates the visual difference between pulp from reamer-type extractors (Brown) and standard squeezer-type extractors (JBT).
5.10.1 Production factors that affect commercial pulp quality
Table 5.1 shows some of the process conditions that have a significant influence on pulp properties.
5.10.2 Process steps in pulp production
The exact configuration of the pulp line will vary from plant to plant. Design will depend on the type of extraction system and processor preference. The basic pulp production steps are shown in Figure 5.23.
Pulp from the primary finishers in juice clarification (rather than pulpy juice from the extractors) is sometimes taken as feed to the pulp production lines. Dilution with juice prior to the defect removal step may then be needed.
Extraction
During the juice extraction process, segment walls and cell sac walls are torn into pieces. Both the reamer-type extractor and the specially designed squeezer-type extractor for premium pulp put less shear force on the pulp compared with the standard squeezer-type extractor. This results in larger and less fragmented pulp pieces. However, defects such as core and seeds also end up in the pulpy juice from the extractors. This imposes greater demands on the defect removal system. When pulp from the primary finishers in juice clarification (rather than pulpy juice) feeds the pulp production line, dilution with juice is usually required before defect removal.
Defect removal
Defects are normally described as small fragments of peel, membrane or seed. The absence of defects in the final product is an important quality parameter, so their removal from the pulp/juice slurry is a priority.
Defects are removed in a series of separation steps. The first step may be a classifying finisher – a paddle-type finisher incorporating screens with large perforations that allow juice and cells to pass through but block large seeds and pieces of membrane. The pulpy juice stream then goes to one or more hydrocyclones. If there are a lot of defects, two or more hydrocyclones are used in series. Hydrocyclones are based on gravity separation and remove defects that have a higher density than the pulp slurry.
Figure 5.24 shows the liquid and particle flow in a cyclone. The infeed, which is tangentially introduced into the cone, starts moving in a downward spiral along the cyclone wall. As it nears the cone outlet, some of the product exits through the underflow orifice while the majority changes direction and flows upward to the cyclone overflow, taking an inner spiral path. If the density of the particles exceeds that of the liquid, the centrifugal force presses the particles against the cyclone wall from where they are pushed down and out through the bottom opening.
Separation in a cyclone improves at lower solids concentration and lower liquid viscosity. Small immature seeds are lighter than pulp slurry and difficult to remove. Thus, the quality of fruit delivered to the processor is important to defect removal performance.
Concentration (primary finishers)
The “cleaned” pulp stream from the defect removal system is normally concentrated prior to heat treatment for two reasons:
- Heating/chilling less product saves energy
- Less juice is subjected to additional heat treatment
Concentration is done in a screw-or paddle-type primary finisher. Paddle finishers treat pulp particles more gently. Finisher operation can be adjusted to ensure the pulp concentration of the discharged pulp slurry is at the required strength for the downstream pasteurization step. In Florida, most processors configure their equipment to achieve a typical pulp concentration of 400-500 g/l in pulp slurry from the primary finisher. In Brazil, plants differ from 150-200 g/l up to 500 g/l pulp concentration. The lower range is due to using plate heat exchangers in the pasteurizer.
The pulp stream from the primary finisher to pasteurization cannot be kept constant. It will vary in both flow rate and pulp concentration (10-15%) during a production shift. Over a season, different fruit varieties and extractor settings will give even wider variations.
Heat treatment
The two objectives of pulp slurry pasteurization are to:
- Inactivate enzymes
- Destroy relevant microorganisms
The necessary degrees of enzyme inactivation and microbial reduction depend on how the pulp will be further processed and stored. The required inactivation level determines the pasteurization conditions (temperature and time). As enzymes in oranges are located in the fruit cell walls, enzyme concentration is significantly higher in pulp slurry than in clarified juice. To achieve complete enzyme inactivation, the heat treatment of pulp slurry needs to be more intensive than of juice. However, complete enzyme inactivation is normally not required. Enzyme activity should be reduced to such an extent that the pulp:
- Is stable during bulk storage
- Will not lead to cloud separation in reconstituted juice.
If the downstream handling of heat-treated pulp is non-aseptic (for instance, it takes place in the drying finisher) 100% destruction of microorganisms is not required. This is the case for pulp stored frozen, the most common storage method. Here, the heat treatment is referred to as “stabilization”. Typical heating conditions are 90-100°C for 30 seconds.
When pulp is to be stored chilled in aseptic bag-in-box containers, heat treatment may be referred to as “pulp stabilization/sterilization”. Temperatures in excess of 100°C are normally used. A higher degree of enzyme inactivation is required for chilled storage compared to frozen storage. Aseptic storage also requires that heat-treated pulp has no microbial activity. Furthermore, downstream equipment must not recontaminate the product.
Which heat exchanger?
The heat exchangers used for pasteurizing pulp slurry are typically of tubular type. Any obstructions on the product side, such as contact points in a plate heat exchanger, should be avoided. Often the heat exchangers incorporate a single product tube. With this type there is no risk of uneven product flow. However, throughput is limited due to the pressure drop.
A multitube heat exchanger (see Figure 7.12) can process high pulp flow rates without the drawback of excessive pressure drops. The inlet to the parallel tubes requires careful design to ensure that pulp does not stick to tube entrances thereby causing blockage and uneven flow rates through the tubes.
Heat treating pulp at concentrations much above 500 g/l is not really feasible in tubular heat exchangers because heat transfer coefficients rapidly decline above this concentration. Efficient heat transfer is inhibited by the product’s high cellulose content. Using tubular heat exchangers for higher pulp concentrations requires very large units, which entails slow heat-up and cool-down times and resultant loss of product quality. A pasteurization system for pulp using multitube heat exchangers can also have the dual function of pasteurizing NFC.
The nature of the pulp recovery process tends to entrain air into the product stream. This has to be considered in the design of the heat treatment process.
Concentration (drying or final finisher)
Traditionally, the heat-treated pulp is further concentrated up to 950-1,000 g/l using a final or drying finisher. Although still wet it is called “dry” pulp because it will not release any free liquid when pressure is applied to it. The residual liquid is mainly adsorbed onto the cellulose membranes.
The concentration of dry pulp is measured for product specification by a special method called Quick Fibre (described in section 8). The liquid in the pulp, essentially NFC, typically corresponds to 5-8% of pulp mass for standard pulp and 9-13% for premium pulp. Thus, when pulp is added during reconstitution at the juice packer the juice still present in the “dry” pulp will provide additional NFC.
Packing in boxes/drums for frozen storage
The concentrated pulp is normally packed in 20 kg corrugated cardboard boxes lined with a polyethylene bag, which are then frozen. Freezing can take several days. Pulp may also be packed in drums (200 l/55 gallon) for frozen storage. However, drums are not often supplied to juice packers as they are usually too large for the batches of reconstituted juice.
The enzyme concentration is higher in pulp slurry than in clarified juice
Packing in aseptic bag-in-box containers for chilled storage
If the stabilization process is modified to become a stabilization/sterilization process, it is possible to pack pulp aseptically and store it refrigerated. Packing is done directly after heat treatment. Hence, the aseptic pulp will be bulk stored at a much lower concentration than frozen pulp.
The disadvantage of packing pulp aseptically at 500 g/l concentration is that larger storage (and shipping) volumes are needed for the same amount of dry pulp. The advantage is that the pulp is much easier to handle as it is pumpable and needs no thawing or crushing. It also makes it possible to enhance the final product. When aseptic pulp is added back to juice reconstituted from concentrate, juice present in the aseptic pulp (effectively NFC) may provide some of the desired flavour associated with NFC products. Aseptic pulp is produced by several processors in Florida.
5.11 Pulp wash production
Pulp washing is carried out to recover juice solubles in pulp supplied by juice finishers and from centrifuges in the clarification or deoiling process. Thorough pulp washing can increase the total yield of soluble solids by 4-7%, which contributes significantly to overall plant economics. The process steps are shown in Figure 5.25.
Juice sugars are reclaimed by a counter-current washing system. The pulp/water slurry is strained through a finisher between the washing stages. The separated “juice” is called pulp wash. Process development includes the use of static mixers to blend water and pulp and increase the recovery of soluble juice and pulp components into the wash stream. The pulp wash is concentrated by evaporation and added back to concentrated orange juice (if the law permits) or used as a base for juice drinks.
Cost-effectiveness dictates the number of stages in a pulp washing system. A maximum of four stages can recover up to 50%, 63%, 75% and 80% respectively of the available juice sugars. The amount recovered depends on the water-to-pulp ratio as well as fruit variety and maturity.
Debittering
Pulp wash is high in limonin, which causes bitterness. Non-treated pulp wash is therefore of limited use as add-back into high quality juice drinks. However, the bitter taste can be removed by a debittering process in which clarified pulp wash passes through a bed of polymeric resin that adsorbs the bitter components.
Pulp and other suspended solids should be removed from the liquid before entering the reactor to prevent blocking of the resin bed. Pulp separation is commonly done using ultrafiltration membranes (0.1-0.01 micro-metres). Sometimes a clarifying centrifuge is installed upstream of the ultrafiltration unit to reduce the load on the membrane filters and increase operating time between filter cleanings. Using a high-efficiency depulping centrifuge with continuous pulp discharge reduces solids to < 1%. The liquid may then flow directly to the debittering reactors without ultrafiltration.
The resin bed has a defined adsorption capacity. When debittering efficiency slows or a high pressure drop has built up, the reactor is stopped for back-flushing and to regenerate the resin bed with caustic solution. Parallel use of two debittering reactors ensures continuous operation: while one reactor is in production, the other is in cleaning/regeneration mode.
After exiting the reactor, the debittered liquid is recombined with the separated pulp stream and sent to evaporators.
Enzyme treatment
The high content of pectin in pulp wash leads to a greater increase in viscosity during evaporation compared with pure juice. This can lead to a limit of 40 °Brix for pulp wash concentration. As a result, the pulp washing process often includes an enzyme treatment step to break down pectin. Typical conditions are a retention time of up to one hour at 45°C in the reactor tank. After centrifugation, enzyme-treated pulp wash can be concentrated to the normal 65 °Brix level and then blended with orange juice concentrate or packed in 200 litre drums and frozen.
Pulp washing can increase the total yield of soluble solids by 4-7%
Washed cells
Washed cells can either be sent to the feed mill or bulk packed in 25 kg cardboard boxes or 200 litre drums, which are stored frozen. The product is traded as washed pulp or washed cells and used in some drink applications.
Regulations for and use of pulp wash
Pulp wash is often used as a source of fruit solids in formulated beverages and juice drinks, and as a clouding agent for providing body and mouthfeel. Pulp wash may be included in orange juice concentrate in Brazil and US provided that it is produced as part of the juice extraction process. As of 2001, also EU regulations permit in-line water extraction of pulp for the production of orange juice concentrate. Likewise, the current fruit juice standards of Codex Alimentarius, issued in 2005, follow the same principles.
Nevertheless, the quality standards and marketing approach of some processors or organizations may still preclude the addition of pulp wash in concentrate. Pulp wash may not be added to NFC.
Pulp wash is also referred to as water-extracted soluble orange solids (WESOS).
5.12 Core wash
The squeezer-type extractors produce a separate stream containing the fruit core of rag, seeds and pulp (see Figure 5.6). As with pulp wash production, water can be added to this mixture to extract soluble solids from the central fruit material. The resulting core wash is very bitter and needs to undergo a debittering process (as described in section 5.11) before it can be used in beverage production.
Core wash “juice” is very opaque and provides high capacity cloud. It is a desirable ingredient for cloudy beverages, both still and carbonated, and replaces synthetic clouding agents such as brominated vegetable oils and ester gum.
5.13 Reduced acid orange juice
Orange juice acidity can be reduced by removing some of the citric acid in the juice. Acid reduction, which is primarily deployed in the US, enables the citrus industry to:
- Serve the growing number of consumers who are sensitive to acid foods and therefore refrain from drinking orange juice
- Use more fruits with a low Brix/acid ratio for juice production
Development of deacidification began in the late 1970s in Florida. In 1992 “reduced acid frozen concentrated orange juice” became an approved juice product in the US and was included in the Federal Standards of Identity. Ten years later, reduced-acid NFC was introduced in the US market.
Elsewhere, regulatory bodies have been more reluctant to accept acid-reduction in orange juice manufacturing. In Europe, orange juice marketed as “low acid” is made from fruit with naturally low acid content.
Deacidification
The deacidification process (see Figure 5.26) is similar to the debittering process in that juice with very low suspended material (<1%) flows through a polymeric resin bed. Citric acid is removed from the juice by ion-exchange, using a weak base anion resin. The resin basically exchanges its hydroxide ions (OH-) for citrate ions (C6H5O7 3-) in the juice, leaving citrate on the resin.
The efficiency of the resin drops as it becomes saturated with citrate. It requires regeneration using a basic solution at regular intervals.
Besides citric acid, orange juice contains highly desirable nutritional acids. Fortunately, the weak base anion resins used in deacidification favour removal of citric acid (being a strong acid) over the weak nutritional acids. Analyses show that ascorbic acid and folic acid content is little influenced by the process.
Juice pH – and microbiological risk – increase as acid is removed. It is therefore important to blend deacidified juice with regular juice directly after the deacidification process to ensure a pH below 4.6.
5.14 Essence recovery
Essence recovery is an integral part of the evaporation process and is described in the section on concentrate production.